Gas Lasers
Definition: lasers with a gas (or plasma) as gain medium
More general term: lasers
More specific terms: ion lasers, helium–neon lasers, helium–cadmium lasers, argon ion lasers, CO2 lasers, CO lasers, excimer lasers, nitrogen lasers, hydrogen lasers, metal vapor lasers
German: Gaslaser
Category: laser devices and laser physics
Author: Dr. Rüdiger Paschotta
Cite the article using its DOI: https://doi.org/10.61835/2ke
Get citation code: Endnote (RIS) BibTex plain textHTML
A variety of lasers is based on gases as gain media. The laser-active entities are either single atoms, ions or molecules, and are often used in a mixture with other substances having auxiliary functions. During operation, the gas is often in the state of a plasma, containing a significant concentration of electrically charged particles.
The operation of a gas laser usually requires a high-voltage supply, but not necessarily with a high electrical power.
While gas lasers have been very important laser sources in the early times, many of them have later been replaced with more compact low-cost laser diodes and partly with solid-state lasers. However, for some laser applications certain types of gas lasers are hard to replace and therefore still widely used. For example, carbon dioxide lasers are still important in laser material processing.
Types of Gas Lasers
Gas lasers can be grouped according to the nature of their laser-active species: neutral atoms, ions or molecules. There is a separate encyclopedia article on metal vapor lasers; examples of those are copper vapor lasers, alkali vapor lasers and helium–cadmium lasers. Excimer lasers are also briefly mentioned here.
Neutral Atom Gas Lasers
Some gas lasers use neutral atoms as the laser-active species:
- Helium–neon lasers (He–Ne lasers), first demonstrated already in 1961, often emit red light at 632.8 nm, but can also be made for other wavelengths such as 543.5 nm (green), 594.1 nm (yellow), 611.9 nm (orange), 3.39 μm, or 1.15 μm by introducing intracavity losses with appropriate spectral characteristics. Typical He–Ne lasers have a gas cell with a length of the order of 20 cm and generate a few milliwatts of output power in continuous-wave operation at 632.8 nm, using several watts of electrical power. Helium–neon lasers are often used for alignment and in interferometers, and compete with laser diodes, which are more compact and efficient. Some He–Ne lasers serve in optical frequency standards.
- Much less common are gas lasers based on other noble gases like argon, krypton and xenon. Helium–xenon lasers can emit e.g. at 2.026 μm or at 3.5 μm.
Common characteristics of neutral atom gas lasers are that they use a glow discharge with relatively low power density and emit only relatively low optical powers (normally far below 1 W), usually in continuous-wave operation. During operation, the gas temperature stays relatively low. The emission wavelengths are mostly in the region between 1 μm and 10 μm, with the notable exception of the helium–neon laser.
Single-frequency operation is normally not difficult to achieve, at least with a relatively short laser tube.
Some metal vapor lasers (e.g. copper vapor) also work with neutral atoms, but with substantially different characteristics: higher power density and typically pulsed operation.
Ion Lasers
Ion lasers typically utilize positively charged ions is the active medium. The most common types of ion lasers are the following:
- Argon ion lasers use a typically about 1 m long water-cooled tube with an argon plasma, made with an electrical discharge with high current density in order to achieve a high degree of ionization. They can generate more than 20 W of output power in green light at 514.5 nm, and less at some other wavelengths such as 457.9, 488.0, or 351 nm. Their power efficiency is fairly low, so that tens of kilowatts of electrical power are required for multi-watt green output, and the cooling system has corresponding dimensions. There are smaller tubes for air-cooled argon lasers, requiring hundreds of watts for generating some tens of milliwatts. Argon ion lasers can be used e.g. for pumping titanium–sapphire lasers and dye lasers, and are rivaled by frequency-doubled diode-pumped solid-state lasers.
- Krypton ion lasers are similar to argon ion lasers and can emit high powers at 647.1 nm and some other wavelengths.
Ion lasers belong to the most important visible gas lasers, and some are even suitable as ultraviolet lasers. In contrast to that, most other gas lasers emit in the infrared.
Common characteristics of ion lasers are that they require excitation with a rather high power density. A sufficiently high gain is normally achieved only in a regime where the output power is at least about 1 W, and several kilowatts of electrical power are consumed. The large amount of waste heat needs to be reduced with an effective laser cooling system.
The high power density and high plasma temperature is unfortunately associated with a relatively limited lifetime of the expensive laser tube – often well below 1000 h. This together with the high electricity consumption leads to rather high operation expenses. Therefore, such lasers have one more been replaced with diode-pumped and frequency-doubled solid-state lasers.
Due to the substantial heating of the tube, a relatively long warm-up time is often required for achieving stable operation.
Single-frequency operation is generally difficult to achieve with ion lasers, since the required long tube length leads to a small free spectral range of the laser resonator, while substantial Doppler broadening of the laser transition impedes narrow-linewidth operation.
Molecular Lasers
Gas lasers can also utilize molecules as laser-active agents. Here, the excited states involve vibrations and rotations of those molecules. The relatively low frequencies of those excitations lead to correspondingly low optical frequencies (long wavelengths) of laser emission.
The most important examples of molecular gas lasers are described in the following:
- Carbon dioxide lasers (CO2 lasers) use a gas mixture of CO2, helium (He), nitrogen (N2), and possibly some hydrogen (H2), water vapor, and/or xenon (Xe) for generating laser radiation mostly at 10.6 μm. They have wall-plug efficiencies above 10% and are suitable for output powers of multiple kilowatts with fairly high beam quality. Some high-power CO2 lasers use a system for quickly circulating the gas (forced convection, fast flow). They are widely used for laser material processing, e.g. cutting, welding and marking, but also in laser surgery.
- Carbon monoxide lasers (CO lasers) can have wall-plug efficiencies of the order of 40%, thus being substantially more power-efficient than CO2 lasers. They can emit on various lines between 4.8 μm and 8.3 μm and are mostly used as light sources for laser absorption spectroscopy. Following technological advances concerning the device lifetime, CO lasers emitting around 5.5 μm might also become interesting for laser material processing (e.g. cutting of glasses); in comparison with the widespread CO2 lasers, they offer better absorption in many materials and better focusing capabilities.
- Nitrogen lasers are another type of pulsed ultraviolet laser, based on pure nitrogen, a nitrogen–helium mixture, and sometimes even simply air (with lower performance). Emission typically occurs at 337.1 nm in the form of short pulses; a self-terminating laser transition is used. The high gain leads to relatively efficient superluminescent emission even without a laser resonator. Nitrogen lasers are relatively easy to build and operate, and have been made by many hobbyists without refined laboratory equipment.
For more details, see the article on molecular lasers.
Common characteristics of molecular gas lasers are long emission wavelengths, high output powers and relatively high power conversion efficiencies, comparing with neutral gas lasers. Due to the low Doppler width of the used layer transitions, it is generally easy to achieve single-frequency operation at least in lower-power versions.
Excimer Lasers
Excimer lasers (rare gas halide lasers, exciplex lasers) are also pumped with an electrical discharge, but in that case the pumping energy is used to form unstable molecules which can emit photons when disassociating. Most excimer lasers are ultraviolet lasers and are operated with current pulses, leading to the emission of intense nanosecond pulses. They are used for various types of material processing, including pulsed laser deposition, laser marking, and the fabrication of fiber Bragg gratings. There are also medical applications e.g. in ophthalmology.
Exotic Types of Gas Lasers
Chemical lasers convert chemical energy of gases into laser light (typically in the mid- or near-infrared region) with powers up to the megawatt level. There are e.g. hydrogen-fluoride (HF) lasers, fueled with H2 and F2, which is converted to HF, and oxygen-iodine lasers (COIL). Chemical lasers are mainly studied for military purposes, e.g. as anti-missile weapons, to be operated even on board large airplanes.
Raman gas lasers are Raman lasers, based on optical amplification via stimulated Raman scattering rather than on stimulated emission of excited ions. They can e.g. use a hydrogen cell, and need to be optically pumped.
A particularly exotic type of gas laser is based on a hollow-core fiber. Here, one utilizes the waveguide function of such a fiber and obtains laser gain from a gas (e.g. acetylene) with which the hollow fiber core is filled. Optical pumping is possible with a laser diode. An example for such a gas fiber laser is an acetylene laser emitting in the spectral region from 3.1 μm to 3.2 μm [14]. Even terahertz lasers can be made in similar ways [15].
Various Technical Aspects of Gas Lasers
Excitation
The usual way of creating a population inversion as the prerequisite for gain via stimulated emission is pumping the gas with an electric discharge. Here, electrons are accelerated in an electric field within the gas, and in collisions with atoms or molecules they transfer energy to them. Through complicated excitation processes, the upper laser level can be populated. Stimulated emission then brings the atoms to a lower state, but typically not the ground state. That final level may be depopulated in different ways, for example by spontaneous emission (e.g. in helium–neon lasers) or by collisions with other atoms or molecules. In some cases (e.g. copper vapor lasers), one has a self-terminating laser transition, which allows only for pulsed operation.
A frequently used method is to primarily excite one species (e.g. helium) in the gas with the electric discharge to some metastable state and exploit an energy transfer process. For example, in helium–neon lasers, the metastable helium atoms transfer energy to neon atoms. By coincidence, the neon atoms have excited states which are only slightly lower in excitation energy than the two dominant metastable states of helium. Therefore, excitation energy can be easily transferred in collisions. From there, the neon atoms are transferred to other levels, which can be used as upper laser levels. A similar mechanism is used in CO2 lasers, where nitrogen (N2) plays a helpful role. The situation is somewhat different in helium–cadmium lasers, where Penning ionization is used: the energy transfer to cadmium is associated with ionization of the cadmium. Here, the freed electron can take away some variable amount of energy, so that the efficiency of the process does not depend on a close match of excitation energies.
The electric field is often a DC field applied through electrodes (e.g. ring electrodes at the ends, transmitting light in the middle), but radiofrequency (RF) excitation is also used frequently.
The used electric discharges a different lasers can have rather different characteristics; parameters like the gas density, the current density and the applied voltage can differ a lot. Many gas lasers work with a glow discharge, exhibiting relatively low current density and power density, while other types have an intense arc discharge where the power density can be orders of magnitude higher.
Another important method has been implemented in gas dynamic CO2 lasers. Here, a combustion brings the gas to very high temperature, but a rapid reduction of temperature is subsequently achieved in a supersonic expansion nozzle. One exploits the fact that energetically lower vibrational states of the CO2 relax faster than higher-lying states, so that a population inversion is obtained.
Less frequently, one uses other excitation mechanisms, for example involving an electron beam, a chemical reaction (→ chemical lasers) or optical pumping (particularly for some metal vapor lasers).
Besides, there are Raman lasers based on Raman-active gases. Here, one exploits an optical nonlinearity rather than classical laser operation.
Cooling
At least in the case of high-power lasers, substantial amounts of heat deposited in the gas need to be removed in order to avoid various possible effects of overheating – for example, the excitation of atoms or molecules into unwanted states, chemical processes or damage of tube walls. Different methods of gas cooling are used:
- In some cases, the heat is removed by diffusion to tube walls or to electrodes, which may be water-cooled. That heat diffusion can be optimized by adding helium to the gas (which sometimes also performs other functions) and by having the cooled tube walls as close to the laser-active volume as possible.
- In other cases, one rapidly pumps the gas through the system – usually in a closed system with a heat exchanger outside the laser-active region.
Regenerating the Gas
In some gas lasers, the laser gas deteriorates over time due to various effects, such as contamination due to sputtering at electrodes or by chemical processes such as dissociation of molecules. (An example for the latter is the transformation of CO2 into CO and O2 in CO2 lasers.) It may also happen that the gas pressure gets reduced over time, e.g. by incorporation of gas constituents into the tube walls.
For such reasons, a consistent performance and long lifetime is sometimes achieved only with additional measures. In some cases, one completely exchanges the laser gas after some number of operational hours. In other cases, one uses a sealed system, not allowing a gas exchange, but adds additional gases or some solid as a catalyzer to undo unwanted chemical processes. For example, CO may be oxidized back to CO2 in a sealed CO2 laser, using H2 and O2 additions to the gas.
Output Power, Beam Quality and Other Characteristics
There are gas lasers are very different types, which greatly differ in various characteristics:
- A wide range of laser transitions can be addressed with different gases, from the ultraviolet through the visible spectral region far into the infrared.
- While some types of gas lasers (e.g. CO2 lasers) can reach output powers of many kilowatts, others are hard to get beyond some tens of milliwatts.
- Most gas lasers emit with a high beam quality, often close to diffraction-limited, since the gas introduces only weak optical distortions, despite considerable temperature gradients.
- Some lasers are particularly suitable for continuous-wave operation, while others are limited to pulsed operation – frequently with nanosecond pulse durations (→ nanosecond lasers).
- The lifetime of a gas laser system can be rather long (many thousand hours of operation); gases are fairly robust and can also often be easily exchanged. However, the lifetime may be limited by other factors such as the erosion of electrodes or the blackening of gas tubes.
Low Density, Low Energy Storage
Gases are laser gain medium with a particularly low density. Even if a solid-state gain medium contains laser-active ions only as a dopant with a small concentration of e.g. 1%, the density of those ions is typically quite high compared to that of gas atoms, ions or molecules.
Nevertheless, gases can produce substantial laser gain, essentially by utilizing allowed laser transitions rather than (weakly) forbidden transitions (as in solid-state lasers).
However, the low density implies that only a quite limited amount of energy can be stored. Therefore because lasers are generally not suitable for generating very intense laser pulses. An exception are CO2 lasers, where the upper-state lifetime is long enough for effective Q switching.
Another consequence of the low density is that the refractive index of the gain medium is close to 1, and not much influenced e.g. by variations of temperature and gas pressure. Therefore, one has very weak thermal lensing, which makes it easier to achieve a high beam quality even at high power levels.
Self-terminating Laser Transitions
Some gas lasers have self-terminating laser transitions, where the lower state has a long lifetime. Lasing stops once the lower-state population becomes too high. Examples of such gas lasers are nitrogen lasers and copper vapor lasers. Excimer lasers can also only be operated in pulsed mode, although for different reasons [9].
More to Learn
Encyclopedia articles:
Suppliers
The RP Photonics Buyer's Guide contains 41 suppliers for gas lasers. Among them:

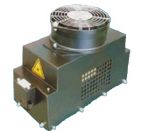
Sacher Lasertechnik
Sacher Lasertechnik offers the following gas lasers:
- air-cooled argon ion laser systems emitting at 458 nm, 488 nm or 514 nm, also multi-line emitters, with up to 500 mW output power
- helium–neon lasers for industrial and scientific applications, emitting up to 20 mW at 633 nm


Edmund Optics
Edmund Optics offers various kinds of gas lasers, e.g. helium–neon lasers from Lumentum with up to 22.5 mW output power and improved stability, used in interferometers and metrology, and Coherent Diamond C-Series CO2 lasers.
Bibliography
[1] | W. R. Bennett, Jr., A. Javan, and D. R. Herriott, “Gas optical maser”, U.S. Patent 3 149 290, 15 September 1964 |
[2] | W. R. Bennett, “Inversion mechanisms in gas lasers”, Appl. Opt. 4 (S1), 3 (1965); https://doi.org/10.1364/AO.4.S1.000003 |
[3] | C. K. N. Patel, “CW high-power N2-CO2 laser,” Appl. Phys. Lett. 7 (1), 15 (1965); https://doi.org/10.1063/1.1754233 |
[4] | W. B. Bridges and A. N. Chester, “Visible and UV laser oscillation at 118 wavelengths in ionized neon and other gases”, Appl. Opt. 4 (5), 573 (1965); https://doi.org/10.1364/AO.4.000573 |
[5] | A. L. Bloom, “Gas Lasers”, Appl. Opt. 5 (10), 1500 (1966); https://doi.org/10.1364/AO.5.001500 |
[6] | A. Javan, W. R. Bennett Jr., and D. R. Herriott, “Population inversion and continuous optical maser oscillation in a gas discharge containing a He–Ne mixture”, Phys. Rev. Lett. 6 (3), 106 (1961); https://doi.org/10.1103/PhysRevLett.6.106 |
[7] | L. E. S. Mathias and J. T. Parker, “Stimulated emission in the band spectrum of nitrogen”, Appl. Phys. Lett. 3 (1), 16 (1963); https://doi.org/10.1063/1.1723557 |
[8] | W. B. Bridges, “Laser oscillation in singly ionized argon in the visible spectrum”, Appl. Phys. Lett. 4, 128 (1964); https://doi.org/10.1063/1.1753995; erratum: Appl. Phys. Lett. 5, 39 (1964); https://doi.org/10.1063/1.1754038 |
[9] | J. Coutts and C.E. Webb, “Stability of transverse self-sustained discharge-excited long-pulse XeCl lasers”, J. Appl. Phys. 59 (3), 704 (1986); https://doi.org/10.1063/1.336586 |
[10] | W. R. Bennett, “Background of an inversion: the first gas laser”, J. Sel. Top. Quantum Electron. 6 (6), 869 (2000); https://doi.org/10.1109/2944.902136 |
[11] | F. Couny et al., “Subwatt threshold cw Raman fiber-gas laser based on H2-filled hollow-core photonic crystal fiber”, Phys. Rev. Lett. 99 (14), 143903 (2007); https://doi.org/10.1103/PhysRevLett.99.143903 |
[12] | B. V. Zhdanov et al., “Rubidium vapor laser pumped by two laser diode arrays”, Opt. Lett. 33 (5), 414 (2008); https://doi.org/10.1364/OL.33.000414 |
[13] | B. V. Zhdanov et al., “Multiple laser diode array pumped Cs laser with 48 W output power”, Electron. Lett. 44 (9), 582 (2008); https://doi.org/10.1049/el:20080728 |
[14] | M. R. A. Hassan, “Cavity-based mid-IR fiber gas laser pumped by a diode laser”, Optica 3 (3), 218 (2016); https://doi.org/10.1364/OPTICA.3.000218 |
[15] | S. Shuai et al., “Optically pumped gas terahertz fiber laser based on gold-coated quartz hollow-core fiber”, Appl. Opt. 58 (11), 2828 (2019); https://doi.org/10.1364/AO.58.002828 |
[16] | O. Svelto, Principles of Lasers, Plenum Press, New York (1998) |
Questions and Comments from Users
Here you can submit questions and comments. As far as they get accepted by the author, they will appear above this paragraph together with the author’s answer. The author will decide on acceptance based on certain criteria. Essentially, the issue must be of sufficiently broad interest.
Please do not enter personal data here; we would otherwise delete it soon. (See also our privacy declaration.) If you wish to receive personal feedback or consultancy from the author, please contact him, e.g. via e-mail.
By submitting the information, you give your consent to the potential publication of your inputs on our website according to our rules. (If you later retract your consent, we will delete those inputs.) As your inputs are first reviewed by the author, they may be published with some delay.
Connect and share this with your network:
Follow our specific LinkedIn pages for more insights and updates: