Photodiodes
Definition: semiconductor devices with a p–n or p–i–n structure for the detection of light
More general term: photodetectors
More specific terms: avalanche photodiodes, Geiger mode photodiodes, lateral effect photodiodes, quadrant photodiodes, p–i–n photodiodes, silicon photodiodes, germanium photodiodes, InGaAs and GaAs photodiodes
German: Photodioden
Categories: photonic devices,
light detection and characterization,
optoelectronics,
optical metrology
Author: Dr. Rüdiger Paschotta
Cite the article using its DOI: https://doi.org/10.61835/8jq
Get citation code: Endnote (RIS) BibTex plain textHTML
Photodiodes are frequently used photodetectors, which have largely replaced the formerly used vacuum phototubes. They are semiconductor devices which contain a p–n junction, and often an intrinsic (undoped) layer between n and p layers. Devices with an intrinsic layer are called p–i–n or PIN photodiodes. Light absorbed in the depletion region or the intrinsic region generates electron–hole pairs, most of which contribute to a photocurrent. The photocurrent can be quite precisely proportional to the absorbed (or incident) light intensity over a wide range of optical powers.

Figure 1 schematically shows the typical design of the photodiode on p–i–n type. Here, one has an intrinsic region between an n-doped and a p-doped region, where most of the electric carriers are generated. Through the electrical contacts (anode and cathode), the generated photocurrent can be obtained. The anode may have a ring shape, so that the light can be injected through the hole. A large active area can be obtained with a correspondingly large ring, but that tends to increase the capacitance, thus reducing the detection bandwidth, and increases the dark current; also, the efficiency may drop if carriers are generated too far from the electrodes.
For a high responsivity of a photodiode, one should have a material with a strong absorption for the optical wavelength of interest. When using a thicker layer for obtaining efficient absorption, one may lose a lot of the generated carriers and therefore still not substantially improve the responsivity.
Some photodiodes are available in the form of photodiode arrays of one-dimensional or two-dimensional kind. Two-dimensional detector arrays, e.g. for use as image sensors, may be realized with photodiodes or with other kinds of photodetectors.
For substantially increased responsivity, one may either use avalanche photodiodes (see below) or phototransistors; these are based on quite different operation principles.
Operation Modes of Photodiodes
Photodiodes can be operated in two very different modes:
- Photovoltaic mode: like a solar cell, the illuminated photodiode generates a voltage which can be measured. However, the dependence of this voltage on the light power is nonlinear (see Figure 2), and the dynamic range is fairly small. Also, the maximum speed is not achieved.


- Photoconductive mode: here, a reverse voltage is applied to the diode (i.e., a voltage in the direction where the diode is not conducting without incident light) and measures the resulting photocurrent. The simplest solution for that reversed-biased mode is based on a voltage source and a load resistor, as shown in Figure 3. The dependence of the photocurrent on the light power can be very linear over six or more orders of magnitude of the light power, e.g. in a range from a few nanowatts to tens of milliwatts for a silicon p–i–n photodiode with an active area of a few mm2. The magnitude of the reverse voltage has nearly no influence on the photocurrent and some influence on the (typically small) dark current (obtained without light). A higher reverse voltage tends to make the response faster but also increases the heating of the device, which can be a problem for high photocurrents.

In the photovoltaic mode (see the line for a 1-kΩ load resistor), the response is nonlinear. In the photoconductive mode, shown here for a simple circuit with a reverse bias applied through a load resistor, a very linear response is achieved. The same holds for a constant reverse bias (not shown).
Even when used in photoconductive mode, photodiodes are usually not understood to be photoconductive detectors, which have a significantly different operation principle.
In the simple circuit according to Figure 3, the magnitude of the bias voltage drops with increasing photocurrent due to the voltage drop at the load resistor. While that has little influence on the linearity, it leads to a charging or discharging of the photodiode's capacitance whenever the incident light intensity changes, so that the detection bandwidth is reduced; it may become RC-limited. That introduces a trade-off between detection bandwidth and responsivity: a high bandwidth requires a small load resistor, which leads to a low responsivity and also a higher noise-equivalent power, which is often limited by thermal noise (Johnson noise) of the load resistor.
In order to avoid that trade-off, one often uses a current amplifier (also called transimpedance amplifier). Such an amplifier, which is typically realized with an operational amplifier (op-amp), keeps the voltage at the diode nearly constant (e.g. near zero, or at some possibly adjustable reverse bias), so that the photodiode's capacitance loses much of its relevance. The residual voltage variations at the photodiode are inversely proportional to the gain of the used operational amplifier. Still, it is good to minimize the input capacitance when requiring a high detection bandwidth; for example, it is better to directly connect a photodiode to the current amplifier, instead of using a long cable connection.
Current amplifiers, which are also available as OEM devices, can also have very good noise properties. The relevant figure is the noise-equivalent input current, which can be well below 1 pA/Hz1/2.
Commercially available laboratory current amplifiers help to make power measurements very flexible by providing many different sensitivity settings, and thus a huge dynamic range with low-noise performance, and also possibly a built-in display, adjustable bias voltage and signal offset, adjustable filters, etc.
Semiconductor Materials
Some photodiode materials and their typical characteristics are:
- silicon (Si): low dark current, high speed, good sensitivity between roughly 400 and 1000 nm (best around 800–900 nm)
- gallium arsenide (GaAs): suitable for visible light, e.g. 400 to 700 nm; can be made very fast (but less sensitive) with low temperature growth, neutron irradiation or ion implantation for carrier lifetimes well below 1 ps
- germanium (Ge): high dark current, slow speed due to large parasitic capacity, good sensitivity between roughly 900 and 1600 nm (best around 1400–1500 nm)
- indium gallium arsenide phosphide (InGaAsP): expensive, low dark current, high speed, good sensitivity roughly between 1000 and 1350 nm (best around 1100–1300 nm)
- indium gallium arsenide (InGaAs): expensive (particularly for large active areas), low dark current, high speed, good sensitivity roughly between 900 and 1700 nm (best around 1300–1600 nm)
The indicated wavelength ranges can sometimes be substantially exceeded by models with extended spectral response.
Key Properties of Photodiodes
The most important properties of photodiodes are:
- the responsivity, i.e., the photocurrent per unit optical power – related to the quantum efficiency, dependent on the wavelength
- the active area, i.e., the light-sensitive area
- the breakdown voltage, setting a limit to the usable bias voltage
- the maximum allowed photocurrent (usually limited by saturation, possibly lower for high bias voltages)
- the dark current (in photoconductive mode, dependent on the bias voltage, important for the detection of low light levels)
- the speed, i.e. the bandwidth (see below), related to the rise and fall time, often influenced by the electric capacitance
Additional quantities may be of interest:
- The typically quite high shunt resistance contributes a small current when a bias voltage is applied. It also contributes some thermal noise current, which in some cases limits the sensitivity.
- The normally small series resistance causes an additional voltage drop proportional to the photocurrent, and can also somewhat contribute to the detection noise.
Bandwidth
The speed (bandwidth) of a photodiode is typically limited either by electrical parameters (capacitance and external resistor) or by internal effects such as carrier transit time in the depletion region. (In some cases, relatively slow diffusion of carriers generated outside the depletion region limits the bandwidth.) The highest bandwidths of tens of gigahertz are usually achieved with small active areas (diameters well below 1 mm) and small absorption volumes. Such small active areas are still practical particularly for fiber-coupled devices, but they limit the photocurrents achievable to the order of 1 mA or less, corresponding to optical powers of ≈ 2 mW or less. Higher photocurrents are actually desirable for suppression of shot noise and thermal noise. (Higher photocurrents increase shot noise in absolute terms, but decrease it relatively to the signal.) Larger active areas (with diameters up to the order of 1 cm) allow for handling of larger beams and for much higher photocurrents, but at the expense of lower speed.
The combination of high bandwidth (tens of gigahertz) and high photocurrents (tens of milliamperes) is achieved in velocity-matched photodetectors, containing several small-area photodetectors, which are weakly coupled to an optical waveguide and deliver their photocurrents into a common RF waveguide structure.
Quantum Efficiency
The quantum efficiency of a photodiode is the fraction of the incident (or absorbed) photons which contribute to the photocurrent. For photodiodes without an avalanche effect, it is directly related to the responsivity <$S$>: the photocurrent is
$$I = S \cdot P = \frac{{\eta e}}{{h\nu }} \cdot P$$with the quantum efficiency <$\eta$>, the electron charge <$e$> and the photon energy <$h\nu$>. The quantum efficiency of a photodiode can be very high – in some cases more than 95% – but varies significantly with wavelength. Apart from a high internal efficiency, a high quantum efficiency requires the suppression of reflections e.g. with an anti-reflection coating.
A higher responsivity (although sometimes at the cost of lower quantum efficiency) can be achieved with avalanche photodiodes. These are operated with a relatively high reverse bias voltage so that secondary electrons can be generated (as in photomultipliers). The avalanche process increases the responsivity, so that noise influences of subsequent electronic amplifiers are minimized, whereas quantum noise becomes more important and multiplication noise is also introduced.
Additional Properties
In some cases, additional properties of photodiodes have to be observed, such as linearity of response over a wide dynamic range, the spatial uniformity of response, or the shape of the dynamic response (e.g. optimized for time domain or frequency domain), or the noise performance.
The noise performance of photodiodes can be very good. For high photocurrents, it can be limited by shot noise, although thermal noise in the electronics is often stronger than that. For the detection of very low light levels (e.g. for photon counting), the dark current can also play a role.
The electronics used in a photodiode-based photodetector can strongly influence the performance in terms of speed, linearity, and noise. As mentioned above, current amplifiers (transimpedance amplifiers) are often a good choice.
Fast Photodiodes
For a particularly high detection bandwidth in the gigahertz region, advanced photodiode designs are used. For example, some devices contain an optical resonator around the thin absorbing section. In that way, one can achieve efficient absorption and thus a high quantum efficiency despite a rather small thickness of the intrinsic region, as is chosen for reducing the drift time.
So-called waveguide photodiodes contain an optical waveguide which confines light along its path through the absorbing region. That region can then again be very thin, and nevertheless one can obtain efficient absorption in a short length. By minimizing the length of the active region, one can also minimize the electrical capacitance and reach a very high bandwidth.
In some cases, the electrode structure is made such that it forms an electrical waveguide, where the electric wave can propagate in parallel with the optical wave in the optical waveguide. Such traveling-wave photodiodes can reach a bandwidth well above 100 GHz.
Some semiconductor materials are intrinsically better suited than others for fast photodiodes. For example, indium gallium arsenide (InGaAs) is particularly suitable because that direct band gap material (in contrast to silicon, for example) exhibits a rather short absorption length, allowing the realization of very thin absorbing layers, in which the photocarriers can be quickly collected. For fast avalanche photodiodes, it is also important to have a low ratio of the impact ionization coefficients for holes and electrons.
Sandwich Detectors
There are so-called sandwich detectors or two-color photodiodes consisting of two (or more) photodiodes in a sequence. The top photodiode is made from the materials with the largest band gap energy and absorbs short-wavelength light while transmitting much of the light with longer wavelengths, which cannot be absorbed. That transmitted light then hits a further photodiode. The ratio of powers detected by the photodiodes depends on the wavelength.
The same principle may also be applied with photodiodes made of the same material because at longer wavelengths (closer to the bandgap) the top photodiode will not absorb all light. One again gets a wavelength-dependent ratio of signals from two photodiodes.
Sandwich detectors may be used for remote temperature measurements, for example, where you uses the ratio of signals from two photodiodes: the higher the temperature, the higher is the relative amount of radiation at shorter wavelengths.
Multi-segment Photodiodes and Photodiode Arrays
Photodiodes are available not only as single-segment detectors. There are dual and quadrant photodiodes, which can be used for precision sensing, and also one-dimensional and two-dimensional photodiode arrays. For more details, see the article on position-sensitive detectors.
A photodiode is sometimes integrated into the package of a laser diode. It may detect some light getting through the highly reflecting back facet, the power of which is proportional to the output power. The signal obtained can be used, e.g., to stabilize the output power, or to detect device degradation.

This encyclopedia is authored by Dr. Rüdiger Paschotta, the founder and executive of RP Photonics AG. How about a tailored training course from this distinguished expert at your location? Contact RP Photonics to find out how his technical consulting services (e.g. product designs, problem solving, independent evaluations, training) and software could become very valuable for your business!
More to Learn
Encyclopedia articles:
Blog articles:
Suppliers
The RP Photonics Buyer's Guide contains 73 suppliers for photodiodes. Among them:


Gentec Electro-Optics
Gentec Electro-Optics offers a great range of power detectors based on silicon or germanium photodiodes for powers up to 750 mW.

CSRayzer Optical Technology
CSRayzer offers different kinds of photodiodes used in high speed, ultra-low light detection, and laser range finding, LIDAR and free space communications.
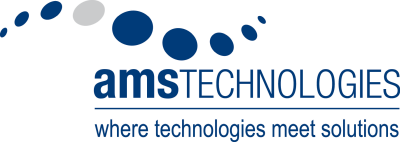

AMS Technologies
AMS Technologies carries an exceptionally broad range of photodiodes (PDs), based on various materials, cooled or uncooled, featuring single devices as well as photodiode arrays or assemblies:
- silicon photodiodes: general-purpose PDs, APDs, high-speed, fast-response or wavelength-enhanced sensitivity variants, duo- and tetra-lateral devices, multi-element PDs and arrays, PD assemblies with filters, TIAs or multiple (sandwich) PDs
- InGaAs photodiodes: general-purpose PDs, PIN, APDs, large active area, segmented or back-illuminated, PD arrays,PD assemblies including PD-amplifier hybrids, mini-DIL optical receivers or pigtails
- GaAs photodiode arrays, GaAs PD/amplifier hybrids
- devices based on HgCdTe, PbS or PbSe
- PD chips based on Si, InGaAs, GaP and AlGaAs

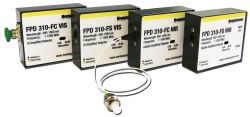
Menlo Systems
Menlo Systems offers a series of photodetectors for lowest light level signals. From avalanche to PIN photodiodes, you can find the detector that is best for your specific application.
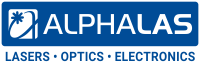
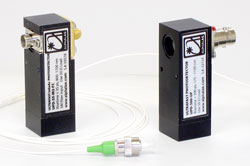
ALPHALAS
Ultrafast photodetectors from ALPHALAS for measurement of optical waveforms with rise times starting from 10 ps and total spectral coverage from 170 to 2600 nm (VUV to IR) have bandwidths from DC up to 30 GHz. Configurations include free-space, fiber receptacle or SM-fiber-pigtailed options and have compact metal housings for noise immunity. The UV-extended versions of the Si photodiodes are the only commercial products that cover the spectral range from 170 to 1100 nm with a rise time < 50 ps. For maximum flexibility, most models are not internally terminated. A 50 Ohm external termination supports the highest speed operation, while a high impedance load generates large amplitude signals. Applications include pulse form and duration measurement, mode beating monitoring and heterodyne measurements. Balanced photodiodes complement the large selection of more than 70 unique models.
Questions and Comments from Users
Here you can submit questions and comments. As far as they get accepted by the author, they will appear above this paragraph together with the author’s answer. The author will decide on acceptance based on certain criteria. Essentially, the issue must be of sufficiently broad interest.
Please do not enter personal data here; we would otherwise delete it soon. (See also our privacy declaration.) If you wish to receive personal feedback or consultancy from the author, please contact him, e.g. via e-mail.
By submitting the information, you give your consent to the potential publication of your inputs on our website according to our rules. (If you later retract your consent, we will delete those inputs.) As your inputs are first reviewed by the author, they may be published with some delay.
Connect and share this with your network:
Follow our specific LinkedIn pages for more insights and updates: