Regenerative Amplifiers
Definition: optical amplifiers with a resonator in which a light pulse can do multiple round trips before being coupled out
More general term: optical amplifiers
German: regenerative Verstärker
Categories: optical amplifiers,
light pulses
Author: Dr. Rüdiger Paschotta
Cite the article using its DOI: https://doi.org/10.61835/2kp
Get citation code: Endnote (RIS) BibTex plain textHTML
A regenerative amplifier is a device which is used for strong amplification of light pulses, usually with ultrashort pulse durations in the picosecond or femtosecond domain (→ ultrafast amplifiers). Multiple passes through the laser gain medium (nearly always a solid-state medium) are achieved by placing the gain medium in an optical resonator, together with an optical switch, which is usually realized with an electro-optic modulator and a polarizer. The number of round trips in the resonator can be controlled with the optical switch, and it can be very large, so that a very high overall amplification factor (gain) is achieved.
Note that although a pulse makes multiple passes in a regenerative amplifier, the term multipass amplifier is often reserved for a device where a fixed number of passes is obtained – not with an optical switch, but just according to the geometric beam path. It would be more natural, though, to consider a regenerative amplifier as one type of multipass amplifier.

Operating Principle of a Regenerative Amplifier
The principle of operation can be understood as follows:
- First, the gain medium is pumped for some time, so that it accumulates some energy.
- Then, the input light pulse is injected into the resonator through a port which is opened for a short time (shorter than the round-trip time) with an electro-optic (or sometimes acousto-optic) switch.
- After that, the pulse can undergo many (typically tens or hundreds) of resonator round trips, being amplified to a high energy level. Typically, one will let the pulse energy grow until a substantial part of the energy is extracted from the gain medium.
- Finally, the pulse is released from the resonator. That can be achieved either with a second electro-optic switch, or with the same one as used for coupling in.
This operation principle makes it possible to achieve very high gain and thus pulse energies in the millijoule range with amplifiers of moderate size, or even higher energies with larger devices. Typical pulse repetition rates are of the order of 1 kHz, although repetition rates of hundreds of kHz are sometimes possible. The highest pulse energies are achieved at lower repetition rates.
Note that the seed laser, normally a mode-locked laser, may have a much higher pulse repetition rate of e.g. 80 MHz; only a small number of the generated seed pulses is used by the amplifier. A separate pulse picker is in principle not required, since the regenerative amplifier itself can “pick” an input pulse when required, but one may want to improve the suppression of additional pulses.
A common gain medium for regenerative amplifiers is Ti:sapphire, which has a broad gain bandwidth and high thermal conductivity. Other gain media, e.g. doped with ytterbium or neodymium, are suitable for diode pumping and have a good energy storage capability due to their long upper-state lifetimes, but the generated pulses are longer (typically hundreds of femtoseconds or more) due to the smaller gain bandwidth.
For high repetition rates (e.g. tens of kilohertz or higher), the amplifier gain medium is usually pumped continuously. For lower repetition rates (particularly for pulse periods well above the upper-state lifetime), pulsed pumping (e.g. with a Q-switched laser) is preferable. A common configuration is to use a frequency-doubled YAG laser to pump a regenerative Ti:sapphire amplifier.
Applications of regenerative amplifiers include laser material processing (e.g. cutting of metals with millijoule pulse energies) and scientific experiments e.g. in high-intensity physics, such as high harmonic generation.
Various Issues
Fast and Accurate Switching
Pulse injection and ejection require the use of a Pockels cell and a Pockels cell driver with a short switching time (well below the resonator round-trip time) and precision. These requirements are substantially more challenging than those for simple Q switching of a laser, for example.
Limited Repetition Rates
The possible output pulse repetition rate may be limited by different factors:
- Frequently, one requires an output pulse energy as high as possible, and needs to have a long pumping time between the application cycles – often of the order of the inverse upper-state lifetime of the gain medium. In extreme cases (with intense pulsed pumping), the repetition rate needs to be further decreased because cooling of the gain medium would otherwise be difficult.
- If low pulse energies are sufficient, one can realize far higher pulse repetition rates. A possible limit then arises from the time for the required number of resonator round trips. However, the round-trip time can be limited to a couple of nanoseconds, so that hundreds of round trips can be done within a microsecond, allowing for a repetition rate of the order of 1 MHz, or more with fewer round trips.
Gain Narrowing
The duration of the amplified pulses may be increased due to the limited gain bandwidth of the amplifier medium, which reduces the pulse bandwidth. This so-called gain narrowing effect may be reduced by inserting a properly designed spectral filter in the amplifier, which makes the net gain spectrum wider and more flat, but reduces the power conversion efficiency.
Chromatic Dispersion and Nonlinearities
At least in femtosecond devices, the chromatic dispersion introduced by the Pockels cell (in addition to that from the gain medium and other components) has to be carefully compensated. Nonlinear effects may also occur; these can be effectively suppressed with the methods of chirped-pulse amplification or divided-pulse amplification. If this is not done, the obtainable pulse energy may be limited by nonlinear effects or even optical damage phenomena.
Influences of Losses
The power conversion efficiency of a regenerative amplifier can be severely reduced by the effect of intracavity losses (particularly in the electro-optic switch). Note that the sensitivity to such losses is particularly high in cases with low round-trip gain because this increases the number of resonator round trips required to achieve a certain overall amplification factor.
Finite Lower-state Lifetime
Regenerative amplifiers can also have a reduced gain and power efficiency due to a finite lower-state lifetime, leading to a significant population in the lower laser level during amplification of a pulse, and thus to reabsorption on the laser transition. That problem occurs primarily in amplifiers for very short pulses, but not with chirped-pulse amplification, where the pulse duration within the amplifier is relatively large.
Satellite Pulses
For some applications, it is important to keep the energy of any satellite pulses very low, i.e. to achieve a high pulse contrast. Such satellite pulses can occur in various ways, e.g. via imperfect switching of the Pockels cell or parasitic reflections in the amplifier. An additional pulse picker may be used to suppress pre- and post-pulses.
Bifurcations
For high pulse repetition rates, it can happen that the pulse amplification process exhibits strong fluctuations. Bifurcations with period doubling can also occur, where the pulse energy first alternates between two values, later four or even eight values. For further increased repetition rates, this can lead into deterministic chaos [8, 9, 12]. Fig 2 shows a numerical example case.

Alternatives to Regenerative Amplifiers
A possible alternative to a regenerative amplifier is a multipass amplifier, where multiple passes (each time with a slightly different propagation direction) are arranged with a set of mirrors. That approach does not require a fast modulator, but becomes complicated (also in terms of alignment) if the number of passes through the gain medium is high.
As a rule of thumb, a multipass amplifier may be more appropriate for very high pulse energies if a limited gain is required ( because the input pulse energy is already relatively high), whereas a regenerative amplifier is more suitable for very high gains.
It is common to combine both approaches: a regenerative amplifier provides the high gain, and a final multipass amplifier stage boosts the energy (→ amplifier chains, ultrafast amplifiers).
Another technique for reaching high pulse energies, although not nearly as high as with regenerative amplifiers, is cavity dumping of a mode-locked laser. That technique is particularly suitable for very high pulse repetition rates of several megahertz.
Case Study
The following case study is available, which discusses some aspects of regenerative amplifiers:
- Simulation of a regenerative amplifier
- We numerically simulate the amplification of ultrashort pulses in a regenerative amplifier. It is interesting to analyze the pulse evolution in a regime where nonlinear effects are strong. For a too high high number of resonator round trips, a bifurcation effect is observed.
More to Learn
Encyclopedia articles:
- multipass amplifiers
- ultrafast amplifiers
- chirped-pulse amplification
- ultrashort pulses
- titanium–sapphire lasers
Blog articles:
Suppliers
The RP Photonics Buyer's Guide contains ten suppliers for regenerative amplifiers. Among them:
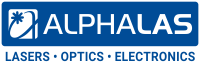
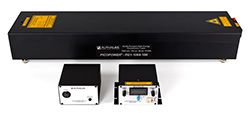
ALPHALAS
Regeneratively amplified picosecond lasers deliver high-energy pulses with durations < 30 ps and > 2 MW peak power at 1064 nm with variable repetition rates from single shot to 10 kHz. They feature a unique synchronization capability with unsurpassed 3.5 ps timing jitter for pulses on demand. Optional wavelengths at 532 nm, 355 nm and 266 nm are available collinearly or as multiple output beams. The power consumption is < 30 W. The air-cooling and compact design attribute to the competitive advantages. Numerous applications include micromachining of metal and non-metal materials, semiconductor wafer inspection, carving, nonlinear optics and ultrafast spectroscopy.
Bibliography
[1] | J. E. Murray and W. H. Lowdermilk, “Nd:YAG regenerative amplifier”, J. Appl. Phys. 51 (7), 3548 (1980); https://doi.org/10.1063/1.328194 |
[2] | W. H. Lowdermilk and J. E. Murray, “The multipass amplifier: theory and numerical analysis”, J. Appl. Phys. 51 (5), 2436 (1980); https://doi.org/10.1063/1.328014 |
[3] | G. Vaillancourt et al., “Operation of a 1-kHz pulse-pumped Ti:sapphire regenerative amplifier”, Opt. Lett. 15 (6), 317 (1990); https://doi.org/10.1364/OL.15.000317 |
[4] | K. Wynne et al., “Regenerative amplification of 30-fs pulses in Ti:sapphire at 5 kHz”, Opt. Lett. 19 (12), 895 (1994); https://doi.org/10.1364/OL.19.000895 |
[5] | C. Horvath et al., “Compact directly diode-pumped femtosecond Nd:glass chirped-pulse-amplification laser system”, Opt. Lett. 22 (23), 1790 (1997); https://doi.org/10.1364/OL.22.001790 |
[6] | S. Biswal et al., “Efficient energy extraction below the saturation fluence in a low-gain low-loss regenerative chirped-pulse amplifier”, J. Sel. Top. Quantum Electron. 4 (2), 421 (1998); https://doi.org/10.1109/2944.686750 |
[7] | J. Kawanaka et al., “30-mJ, diode-pumped, chirped-pulse Yb:YLF regenerative amplifier”, Opt. Lett. 28 (21), 2121 (2003); https://doi.org/10.1364/OL.28.002121 |
[8] | J. Dörring et al., “Period doubling and deterministic chaos in continuously pumped regenerative amplifiers”, Opt. Express 12 (8), 1759 (2004); https://doi.org/10.1364/OPEX.12.001759 |
[9] | D. Müller et al., “Picosecond thin-disk regenerative amplifier”, Proc. SPIE 5120, 281 (2004); https://doi.org/10.1117/12.515524 |
[10] | I. Matsushima et al., “10 kHz 40 W Ti:sapphire regenerative ring amplifier”, Opt. Lett. 31 (13), 2066 (2006); https://doi.org/10.1364/OL.31.002066 |
[11] | M. Larionov et al., “High-repetition-rate regenerative thin-disk amplifier with 116 μJ pulse energy and 250 fs pulse duration”, Opt. Lett. 32 (5), 494 (2007); https://doi.org/10.1364/OL.32.000494 |
[12] | M. Grishin et al., “Dynamics of high repetition rate regenerative amplifiers”, Opt. Express 15 (15), 9434 (2007); https://doi.org/10.1364/OE.15.009434 |
[13] | X. Zhang et al., “Multi-microjoule, MHz repetition rate Ti:sapphire ultrafast regenerative amplifier system”, Opt. Express 20 (7), 7015 (2012); https://doi.org/10.1364/OE.20.007015 |
[14] | E. Caracciolo et al., “28-W, 217 fs solid-state Yb:CAlGdO4 regenerative amplifiers”, Opt. Lett. 38 (20), 4131 (2013); https://doi.org/10.1364/OL.38.004131 |
[15] | H. Fattahi et al., “High-power, 1-ps, all-Yb:YAG thin-disk regenerative amplifier”, Opt. Lett. 41 (6), 1126 (2016); https://doi.org/10.1364/OL.41.001126 |
[16] | M. Ueffing et al., “Direct regenerative amplification of femtosecond pulses to the multimillijoule level”, Opt. Lett. 41 (16), 3840 (2016); https://doi.org/10.1364/OL.41.003840 |
[17] | P. Mason et al., “Generation of Joule-level green bursts of nanosecond pulses from a DPSSL amplifier”, Opt. Express 31 (12), 19510 (2023), [DOI:10.1364/OE.492263] |
[18] | R. Paschotta, case study on a regenerative amplifier |

This encyclopedia is authored by Dr. Rüdiger Paschotta, the founder and executive of RP Photonics AG. How about a tailored training course from this distinguished expert at your location? Contact RP Photonics to find out how his technical consulting services (e.g. product designs, problem solving, independent evaluations, training) and software could become very valuable for your business!
Questions and Comments from Users
Here you can submit questions and comments. As far as they get accepted by the author, they will appear above this paragraph together with the author’s answer. The author will decide on acceptance based on certain criteria. Essentially, the issue must be of sufficiently broad interest.
Please do not enter personal data here; we would otherwise delete it soon. (See also our privacy declaration.) If you wish to receive personal feedback or consultancy from the author, please contact him, e.g. via e-mail.
By submitting the information, you give your consent to the potential publication of your inputs on our website according to our rules. (If you later retract your consent, we will delete those inputs.) As your inputs are first reviewed by the author, they may be published with some delay.
Connect and share this with your network:
Follow our specific LinkedIn pages for more insights and updates: