Erbium-doped Fiber Amplifiers
Acronym: EDFA
Definition: fiber amplifiers based on erbium-doped optical fibers
More general term: fiber amplifiers
German: Erbium-dotierte Faserverstärker
Categories: fiber optics and waveguides,
photonic devices,
optical amplifiers,
lightwave communications
Author: Dr. Rüdiger Paschotta
Cite the article using its DOI: https://doi.org/10.61835/jlr
Get citation code: Endnote (RIS) BibTex plain textHTML
Erbium-doped fiber amplifiers are the by far most important fiber amplifiers in the context of long-range optical fiber communications; they can efficiently amplify light in the 1.5-μm wavelength region, where silica-based telecom fibers have their loss minimum. The core element of a fiber amplifier is a piece of rare-earth-doped fiber, which can provide laser amplification via stimulated emission when it is optically pumped with other light injected into the fiber. See the article on fiber amplifiers for information on more general aspects.
Setup and Operation Principle
A typical setup of a simple erbium-doped fiber amplifier (EDFA) is shown in Figure 1. Its core component is the erbium-doped optical fiber, which is typically a single-mode fiber (or sometimes a few-mode fiber).
In the shown case, the active fiber is “pumped” with light from two laser diodes (bidirectional pumping), although unidirectional pumping in the forward or backward direction (co-directional and counter-directional pumping) is also very common. The pump light, which most often has a wavelength around 980 nm or sometimes around 1450 nm, excites the erbium ions (Er3+) into the 4I13/2 state (in the case of 980-nm pumping via 4I11/2), from where they can amplify light in the 1.5-μm wavelength region via stimulated emission back to the ground-state manifold 4I15/2. (See also Figure 1 in the article on erbium-doped laser gain media.)
The setup shown also contains two “pig-tailed” (fiber-coupled) Faraday isolators. The isolator at the input prevents light originating from amplified spontaneous emission from disturbing any previous stages, whereas that at the output suppresses lasing (or possibly even destruction) if output light is reflected back to the amplifier. When used without optical isolators, fiber amplifiers can be sensitive to back-reflections.

Two laser diodes (LDs) provide the pump power for the erbium-doped fiber. The pump light is injected via dichroic fiber couplers. Pig-tailed optical isolators reduce the sensitivity of the device to back-reflections.
Various other components can also be contained in a commercial fiber amplifier. For example, there can be fiber couplers and photodetectors for monitoring optical power levels, pump laser diodes with control electronics and gain flattening filters for a wider gain bandwidth (e.g. C + L band). For particularly compact packages, various passive optical components can be combined into a photonic integrated circuit (planar lightwave circuit).
For low-power EDFAs, one often uses erbium-doped fibers having an effective mode area which is substantially smaller than that of passive telecom single-mode fibers. That could in principle lead to high splice losses. However, the high alumina content of such fibers leads to substantial diffusion during the splicing process, effectively generating a kind of mode field adapter, so that the splice losses can actually be quite small – far smaller than with mechanical splicing.
Very high signal gains, as required e.g. for the amplification of ultrashort pulses to high energies, are usually realized with amplifier chains, consisting of several amplifier stages with additional optical elements (e.g. isolators, filters, or modulators) in between.
Fiber Amplifiers
You can learn about rare earth ions, how to calculate optical powers and ionic excitations in amplifiers, and on many other topics: ASE, forward vs. backward pumping, double-clad fibers, amplification of light pulses, amplifier noise, and multi-stage amplifiers.
Gain Spectrum
The shape of the erbium gain spectrum depends on the absorption and emission cross-sections, which substantially depend on the used host glass of the fiber core. Also, the spectral shape of the gain and not only its magnitude is substantially influenced by the average degree of excitation of the erbium ions because these have a quasi-three-level transition. Figure 2 shows data for a common type of glass, which is some variant of silica with additional dopants e.g. to avoid clustering of erbium ions. Other glass compositions can lead to substantially different gain spectra.

Strong three-level behavior (with transparency reached only for > 50% excitation) occurs at 1535 nm. In that spectral region, the unpumped fiber exhibits substantial absorption losses, but the high emission cross-section allows for a high gain for strong excitation. At longer wavelengths (e.g. 1580 nm), a lower excitation level is required for obtaining gain, but the maximum gain is smaller.
The maximum gain typically occurs in the wavelength region around 1530–1565 nm (the telecom C band). The 1530-nm gain maximum peak is most pronounced for high excitation levels, whereas low excitation levels lead to gain maxima at longer wavelengths. The local excitation level depends on the emission and absorption cross-sections and on the pump and signal intensity (apart from that of ASE light). The average excitation level over the whole fiber length, as is relevant for the net gain spectrum, depends on the pump and signal powers, but also on the fiber length and the erbium concentration. Such parameters (together with the choice of glass composition) are used to optimize an EDFA for sufficiently high and spectrally flat gain in a particular wavelength region, such as the telecom C or L band. L band amplifiers generally work with a low degree of erbium excitation and are designed for a longer cut-off wavelength.
The ease of making a well working EDFA for the telecom C band is the primary reason why this became quite popular. However, optimization for other bands is possible, and allowed a substantial extension of the overall usable wavelength range.
A good flatness of the gain in a wide wavelength region (→ gain equalization), as required e.g. for wavelength division multiplexing (see below), can be obtained by using optimized glass hosts (e.g. telluride or fluoride fibers, or some combination of amplifier sections with different glasses) or by combination with appropriate optical filters, such as long-period fiber Bragg gratings. The latter approach can be realized with a tailored long-period fiber Bragg grating, but at the expense of reduced output power and efficiency.

Case Study: Erbium-doped Fiber Amplifier for Multiple Signals
We optimize an amplifier for equal output powers of signals spanning a substantial wavelength range. There is a trade-off between power efficiency and noise performance.
Erbium-doped Amplifiers in Telecom Systems
After the first demonstration of an erbium-doped fiber amplifier in 1987 [1], it took only five years until commercial amplifier products became available. This is because the benefits for optical fiber communications were easily recognized as being substantial. In many years of further research and development, no viable alternative has been found which could widely replace erbium-doped fiber amplifiers.
EDFAs can serve various functions in systems for telecom systems; the most important applications are the following:
- The power of a data transmitter may be boosted with a high-power EDFA before entering a long fiber span, or a device with large losses, such as a fiber-optic splitter. Such splitters are widely used e.g. in cable-TV systems, where a single transmitter is used to deliver signals into many fibers.
- A fiber amplifier may also be used in front of a data receiver, if the arriving signal is weak. Despite the introduction of amplifier noise, this can improve the signal-to-noise ratio and thus the possible data transmission rate, since the amplifier noise may be weaker than the input noise of the receiver. It is more common, however, to use avalanche photodiodes, which have some built-in signal amplification.
- In-line EDFAs are used between long spans of passive transmission fiber. Using multiple amplifiers in a long fiber-optic link has the advantage that large transmission losses can be compensated without (a) letting the optical power drop to too low levels, which would spoil the signal-to-noise ratio, and (b) without transmitting excessive optical powers at other locations, which would cause detrimental nonlinear effects due to the unavoidable fiber nonlinearities. Many of these in-line EDFAs are operated even under difficult conditions, e.g. on the ocean floor, where maintenance would be hardly possible.
- Although data transmitters are normally not based on erbium-doped devices, an EDFA can be part of equipment for testing transmission hardware. It can also be used in the context of optical signal processing.
These functions can be realized in the telecom C and L bands. Other types of fiber amplifiers, e.g. based on praseodymium, have been considered for other bands, but none can compete with erbium-based devices in terms of gain and gain efficiency.
A particular attraction of EDFAs is their large gain bandwidth, which is typically tens of nanometers and thus actually more than enough to amplify data channels with the highest data rates without introducing any effects of gain narrowing. A single EDFA may be used for simultaneously amplifying many data channels at different wavelengths within the gain region; this technique is called wavelength division multiplexing. Before such fiber amplifiers were available, there was no practical method for amplifying all channels e.g. between long fiber spans of a fiber-optic link: one had to separate all data channels, detect and amplify them electronically, optically resubmit and again combine them. The introduction of fiber amplifiers thus brought an enormous reduction in the complexity, along with a corresponding increase in reliability. Very long lifetimes are possible by using redundant down-rated pump diodes.
Most erbium-doped fiber amplifiers are based on single-mode fiber. However, other types of fiber amplifiers have recently been developed, which can be used in the context of space division multiplexing. Here, the fiber is either a multi-core fiber or a few-mode fiber. Such amplifiers need to be optimized such that the gain in the different cores, or in the second case for the different fiber modes, is quite similar.
The only competitors to erbium-doped fiber amplifiers in the 1.5-μm region are Raman amplifiers, which profit from the development of higher power pump lasers. Raman amplification can also be done in the transmission fiber. Nevertheless, EDFAs remain very dominant.

Case Study: Erbium-doped Fiber Amplifier for Multiple Signals
We optimize an amplifier for equal output powers of signals spanning a substantial wavelength range. There is a trade-off between power efficiency and noise performance.

Case Study: Erbium-doped Fiber Amplifier for a Long-wavelength Signal
Amplified spontaneous emission (ASE) turns out to be a limiting factor, requiring a dual-stage amplifier design.
Further Technical Details
The most common pump wavelength for EDFAs is around 980 nm. Light at this wavelength pumps erbium ions from their ground-state manifold 4I15/2 to the 4I11/2 manifold, from where there is a quick non-radiative transfer to the upper laser level 4I13/2. Due to that quick transfer, there is essentially no deexcitation via stimulated emission by pump light, and very high excitation levels can be achieved. Therefore, this approach makes it possible to achieve the highest gain efficiency (order of 10 dB/mW) and the lowest noise figure, although the power efficiency is not ideal due to the significant quantum defect.
A higher power efficiency can be achieved by in-band pumping around 1450 nm. However, stimulated emission by pump light then limits the achievable excitation level, hence also the gain per unit length, and the maximum gain occurs at longer wavelengths. The noise figure will also be higher.
In high-gain amplifiers, amplified spontaneous emission (ASE) is often the limiting factor for the achievable gain. Due to the quasi-three-level nature of the erbium ions, ASE powers can be different between forward and backward direction, and the maximum ASE can occur at a wavelength which differs from that of maximum gain.
The noise figure of an optimized EDFA is slightly larger than the theoretical limit of 3 dB for a high-gain amplifier; this is mainly due to the quasi-three-level nature. Relatively low-noise performance can be achieved with suitable amplifier design, taking into account particularly the erbium excitation level near the signal input end, which can be strongly influenced by the pump direction.
Various aspects of erbium-doped amplifiers (magnitude and spectral shape of the gain, power conversion efficiency, gain saturation effect, ASE, sensitivity to back-reflections etc.) can be analyzed with suitable fiber simulation software. The resulting quantitative understanding can be the basis for optimization of devices in terms of performance and required components. Generally, the operation details are too complex for optimization based on trial and error.
Dynamic Behavior of EDFAs
Due to the relatively small transition cross-sections of Er3+, particularly in the usual glass hosts, the saturation energy of an EDFA is relatively large: it is easily several microjoules even for a small effective mode area, and tens of microjoules for fibers with large mode area.
An important consequence for telecom amplifiers is that the amplifier gain stays quite precisely constant when amplifying a bit stream, as a energy per bit usually stays orders of magnitude below the saturation energy. (This is different for semiconductor optical amplifiers, having far lower saturation energies.) Only over thousands or millions of data symbols, the gain adjusts itself to the average signal power level.
The upper-state lifetime is rather long (order of 10 ms), implying that the gain reacts quite slowly to any changes in output power. After turning it on, it can take tens of milliseconds to reach the steady state, except in cases with high signal power and accordingly strong gain saturation.
These characteristics are quite useful for amplifying energetic pulses. An EDFA can be pumped over 10 ms, for example, then storing substantial energy, and can then transfer much of that energy to a single signal pulse, reaching a pulse energy of many microjoules or in extreme cases even tens of millijoules. Performance limitations often result mostly from fiber nonlinearities rather than those related to energy storage and amplification.


Case Study: Erbium-doped Fiber Amplifier for Rectangular Nanosecond Pulses
We deal with deformations of the pulse shape due to gain saturation. These can be minimized by pre-distorting the input pulses.
Variants and Other Applications of Erbium-doped Fiber Amplifiers
A high gain in a shorter length can be achieved with ytterbium-sensitized fibers, i.e., fibers doped with erbium and ytterbium. They are also called Er:Yb:glass fibers or ytterbium-codoped fibers. Their concentration of Yb3+ ions is an order of magnitude higher than that of Er3+. The ytterbium ions may be excited e.g. with 975-nm pump light (or even at longer wavelengths such as 1064 nm) and transfer their energy to erbium ions. For a proper choice of the material composition of the fiber core (often with substantial amounts of phosphorus), the energy transfer can be fairly efficient. However, the use of pure erbium-doped fibers is more common in the telecom area because ytterbium sensitization has no essential advantages here and possibly leads to a reduced gain bandwidth due to the modified chemical composition.
Erbium-doped double-clad fibers can be used for generating very high output powers of tens of watts or even more. Ytterbium codoping is in that case usually used because the pump absorption efficiency would otherwise be poor. Even for moderately high signal power levels around 1 to 2 W, as needed e.g. for cable TV (CATV) amplifiers, double-clad Er/Yb amplifiers are used, since suitable pump diodes for core pumping would not be available.
It is also possible to amplify ultrashort pulses in the 1.5-μm region to relatively high energies, using EDFAs in the form of amplifier chains. One exploits the relatively high saturation energy of such amplifiers, particularly when using erbium-doped large mode area fibers.
More to Learn
- Case Study: Erbium-doped Fiber Amplifier for Multiple Signals
- Case Study: Erbium-doped Fiber Amplifier for a Long-wavelength Signal
- Case Study: Erbium-doped Fiber Amplifier for Rectangular Nanosecond Pulses
Encyclopedia articles:
- fiber amplifiers
- optical amplifiers
- rare-earth-doped fibers
- erbium-doped laser gain media
- optical fiber communications
Suppliers
The RP Photonics Buyer's Guide contains 45 suppliers for erbium-doped fiber amplifiers. Among them:

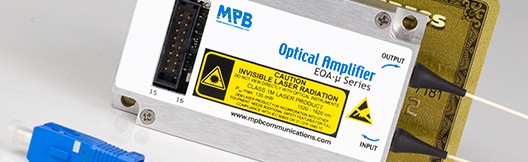
MPB Communications
In 1995, MPB Communications provided their first generation of high-power boosters and quantum-limited noise-figure EDFAs for long-haul, unrepeatered telecom systems.
Today, our EDFA technology can be found in our line of network-ready telecom solutions, as well as in our extensive portfolio of gain modules – which includes ultra-compact amplets with low noise, high gain and low power consumption, but also full-size high power, low noise EDFAs.
High reliability combined with the ease of customization to fit client specific requirements make MPBC EDFAs the ideal choice for your demanding application.
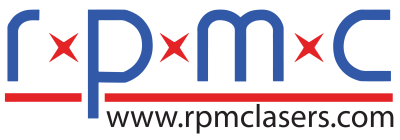
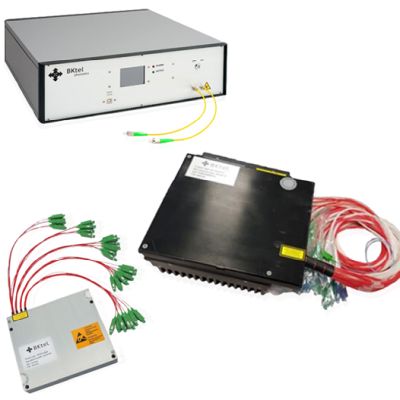
RPMC Lasers
Serving North America, RPMC offers a wide range of Telcordia grade erbium and ytterbium fiber lasers and amplifiers that are deployed in a wide range of applications, including LIDAR, mapping, 3D scanning and telecommunications. The BKtel suite of lasers and amplifiers are available in a range of wavelengths from 1030 nm to 2054 nm and with average powers up to 100 W. Standard and custom options available. Let RPMC help you find the right laser today!

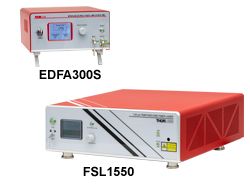
Thorlabs
Within its optical amplifier selection, Thorlabs designs and manufactures both polarization-maintaining (PM) and single mode (SM) erbium-doped fiber amplifiers (EDFAs) for use in the C-Band (1530 – 1565 nm). These benchtop fiber amplifiers join our femtosecond all-PM-fiber erbium-doped amplified oscillator, the FSL1550, which produces <40 fs pulses and provides record peak pulse power.
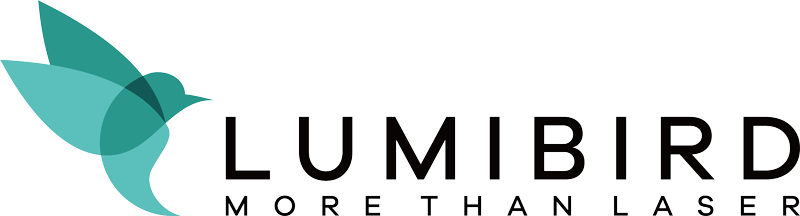

Lumibird
Lumibird manufactures a unique range of CW and pulsed Erbium-Doped Fiber Amplifiers (EDFAs), available as turnkey benchtop instruments or in OEM modules.

CSRayzer Optical Technology
CSRayzer offers erbium-doped fiber amplifier modules, which are suitable for multi-channel operation in the C band, L band or C+L bands. Modules with polarization-maintaining fiber or for pulse amplification are also available.


Menlo Systems
Menlo Systems' erbium-doped fiber amplifiers use polarization maintaining (PM) components only, ensuring excellent stability and low-noise operation. Both, our scientific and OEM platform offer highest average output power and short pulse operation.
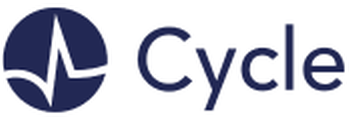
Cycle
Based on Cycle's own femtosecond fiber lasers, the company also offers fiber-based amplifiers (EDFA) with a center wavelength of 1550 nm to 1700 nm. This variant of the SOPRANO-15 is a very attractive solution to amplify the output of beam arrival monitors in synchrotron or FEL facilities, for example. Other custom-made fiber amplifiers are available upon request.
Bibliography
[1] | R. J. Mears, L. Reekie, M. Jauncey, and D. N. Payne, “Low-noise erbium-doped fiber amplifier operating at 1.54 μm”, Electron. Lett. 26, 1026 (1987); https://doi.org/10.1049/el:19870719 |
[2] | E. Desurvire, J. R. Simpson, and P. C. Becker, “High-gain erbium doped traveling wave fiber amplifier”, Opt. Lett. 12 (11), 888 (1987); https://doi.org/10.1364/OL.12.000888 |
[3] | R. I. Laming et al., “Efficient pump wavelengths of erbium-doped optical fiber amplifier”, Electron. Lett. 25, 12 (1989); https://doi.org/10.1049/el:19890009 |
[4] | E. Desurvire et al., “Gain dynamics of erbium-doped fiber amplifiers”, Proc. SPIE 1171, 103 (1989); https://doi.org/10.1117/12.963143 |
[5] | A. A. M. Saleh et al., “Modelling of gain in erbium-doped fiber amplifiers”, IEEE Photon. Technol. Lett. 2 (10), 714 (1990); https://doi.org/10.1109/68.60769 |
[6] | B. Pedersen et al., “Experimental and theoretical analysis of efficient erbium-doped fiber power-amplifiers”, IEEE Photon. Technol. Lett. 3, 1085 (1991); https://doi.org/10.1109/68.118009 |
[7] | B. J. Ainslie, “A review of the fabrication and properties of erbium-doped fibers for optical amplifiers”, J. Lightwave Technol. 9 (2), 220 (1991); https://doi.org/10.1109/50.65880 |
[8] | C. R. Giles and E. Desurvire, “Modeling erbium-doped fiber amplifiers”, IEEE J. Lightwave Technol. 9 (2), 271 (1991); https://doi.org/10.1109/50.65886 |
[9] | M. Yamada et al., “Gain-flattened tellurite-based EDFA with a flat amplification bandwidth of 76 nm”, IEEE Photonics Technol. Lett. 10 (9), 1244 (1998); https://doi.org/10.1109/68.705604 |
[10] | C. Jiang et al., “Improved gain performance of high concentration Er3+–Yb3+-codoped phosphate fiber amplifier”, IEEE J. Quantum Electron. 41 (5), 704 (2005); https://doi.org/10.1109/JQE.2005.845355 |
[11] | X. Zhu and R. Jain, “Watt-level Er-doped and Er-Pr-codoped ZBLAN fiber amplifiers at the 2.7–2.8 μm wavelength range”, Opt. Lett. 33 (14), 1578 (2008); https://doi.org/10.1364/OL.33.001578 |
[12] | G. C. Valley, “Modeling cladding-pumped Er/Yb fiber amplifiers”, Opt. Fiber Technol. 7, 21 (2001) (useful review on amplifier modeling); https://doi.org/10.1006/ofte.2000.0351 |
[13] | K. S. Abedin et al., “Amplification and noise properties of an erbium-doped multicore fiber amplifier”, Opt. Express 19 (17), 16715 (2011); https://doi.org/10.1364/OE.19.016715 |
[14] | Y. Jung et al., “First demonstration and detailed characterization of a multimode amplifier for space division multiplexed transmission systems”, Opt. Express 19 (26), B952 (2011); https://doi.org/10.1364/OE.19.00B952 |
[15] | H. Chen et al., “Integrated cladding-pumped multicore few-mode erbium-doped fibre amplifier for space-division-multiplexed communications”, Nature Photonics 10, 529 (2016); https://doi.org/10.1038/nphoton.2016.125 |
[16] | S. Jain et al., “32-core erbium/ytterbium-doped multicore fiber amplifier for next generation space-division multiplexed transmission system”, Opt. Express 25 (26), 32887 (2017); https://doi.org/10.1364/OE.25.032887 |
[17] | E. Desurvire, Erbium-Doped Fiber Amplifiers: Principles and Applications, John Wiley & Sons, New York (1994) |
[18] | RP Photonics, several case studies on an erbium-doped fiber amplifiers |

This encyclopedia is authored by Dr. Rüdiger Paschotta, the founder and executive of RP Photonics AG. How about a tailored training course from this distinguished expert at your location? Contact RP Photonics to find out how his technical consulting services (e.g. product designs, problem solving, independent evaluations, training) and software could become very valuable for your business!
Questions and Comments from Users
2020-06-15
What is the limitation on minimum optical input power to an EDFA? Would it be possible to achieve very high gain (with multiple common amplifiers) with a very low level optical input (e.g. −60 dBm) at the cost of an increased noise figure?
The author's answer:
The practical limit is usually set by ASE within the required amplification bandwidth. If you need to gain over the full bandwidth of an EDFA, the ASE output already becomes fairly strong around 40 dB, so that it causes a major power loss (in addition to contaminating the signal). Within a narrow bandwidth, you could have more gain, and limit the ASE output power by using narrowband bandpass filters between the amplifier stages. However, it depends on the end on how much ASE contamination of the signal you can tolerate in your application.
Note that the noise figure of the amplifier chain may still be quite low, if the preamplifier stage is well designed; that does not imply low ASE, however, in such a situation with high gain.
2020-09-08
What determines the maximum total output power of an EDFA?
The author's answer:
This mostly depends on the used optical pump power. Besides, it depends on the efficiency, which is mostly determined by details of the active fiber and design details like fiber length, pump wavelength, etc.
2020-09-08
What is the length of erbium-doped fiber in a common amplifier?
The author's answer:
A few meters.
2020-12-10
Why does an EDFA have a limited gain bandwidth, typically covering the C-band?
The author's answer:
This is because its light amplification is based on stimulated emission on a transition between two energy levels (more precisely: Stark level manifolds). Stimulated emission can occur only if the photon energy fits to the energy difference between the two levels.
2020-12-29
Why are erbium-doped fiber amplifiers for the L band typically longer than those for C band?
The author's answer:
The emission cross-sections in the L band are substantially smaller than those for the C band, so that a relatively long length of fiber is required for a certain amplifier gain.
2022-03-17
In figure 2, what is the excitation wavelength, or doesn't it matter?
The author's answer:
It does not matter which wavelength you use for getting a certain amount of erbium excitation. However, the required optical power will depend on the wavelength, and also the maximum amount of excitation you can reach.
2022-09-24
What is typical maximum bandwidth of an EDFA centered at 1550nm?
The author's answer:
This can vary a lot, depending on what type of erbium-doped fiber is used, whether there is a gain flattening filter, and even how you define the bandwidth – maybe the range in which the gain does not deviate more than 1 dB or 3 dB from its nominal value.
2022-10-20
Is the output power divided among wavelengths? Example: max. output 10 watts, beam with 10 wavelengths in. Output 1 watt per wavelength?
The author's answer:
Sure, you couldn't get 10 W each out for 10 signals. The total output power is limited e.g. by available pump power, and possibly by other factors.
2022-11-28
How much will the phase of the signal be changed due to the amplification in an EDFA?
The author's answer:
If you mean the optical phase, that will change a lot, since the EDFA is orders of magnitude longer than a wavelength, and that phase change is also quite sensitive to temperature, mechanical noise etc. However, that phase change is usually not relevant for applications.
2023-03-30
Why does the gain reduce after a certain amplifier length? Is it somehow connected with inversion level?
The author's answer:
Many amplifiers are end-pumped. The pump power is then decaying mostly due to pump absorption. That results in a decreasing excitation level and thus reduced local gain.
2023-04-27
Is there input signal level that will damage an EDFA? Looking at various datasheets, a maximum input power is rarely listed.
The author's answer:
This is because it is hard to destroy such an amplifier with excessive input power. Well, it may work with intense input light pulses, e.g. leading to catastrophic nonlinear self-focusing, but that would be far from the usual operation conditions. It is only that the amplifier gain will drop, see the article on gain saturation.
2024-01-20
What's the difference between pulsed and continuous-wave EDFAs?
The author's answer:
These are essentially the same kinds of devices, but an EDFA may be optimized for operation with pulses. For example, one may want to use an active fiber with larger mode area for increasing the saturation energy or for mitigating nonlinear effects.
Here you can submit questions and comments. As far as they get accepted by the author, they will appear above this paragraph together with the author’s answer. The author will decide on acceptance based on certain criteria. Essentially, the issue must be of sufficiently broad interest.
Please do not enter personal data here; we would otherwise delete it soon. (See also our privacy declaration.) If you wish to receive personal feedback or consultancy from the author, please contact him, e.g. via e-mail.
By submitting the information, you give your consent to the potential publication of your inputs on our website according to our rules. (If you later retract your consent, we will delete those inputs.) As your inputs are first reviewed by the author, they may be published with some delay.
2020-03-17
If I have a laser with very narrow linewidth, how will the EDFA affect the amplified laser linewidth?
The author's answer:
Normally, it will not at all increase the linewidth, despite some addition of amplifier noise. This is essentially because although the amplifier may introduce some time-dependent phase changes, these are limited in extent – no unbounded phase drifts are possible.